INTRODUCTION
I have developed the following information on Metabolic Health for my own
reference and use, but it is available for anybody
interested in Insulin Resistance / Diabetes etc.
I have included many scientific papers here and they mostly speak for themselves ie. I have made no value judgements or made any recommendations.
I am only concerned about Insulin Resistance, Pre-Diabetes and Type 2 Diabetes, meaning Metabolic Health
I am not a Doctor and do not warrant or assume any legal liability or
responsibility for their accuracy, completeness, or usefulness. If you have health concerns see a Doctor.
I apologise for the fact that this study is highly plagerized and thus sort of disjointed as I switch from one source to another.
I have provided many good sources below and some that can be problematic as they are considering just one aspect of the diabetes conundrum.

Insulin resistance is a major feature of Metabolic Health. Insulin resistance is associated with Hyperglycemia, obesity, hypertension, dyslipidemia,
nonalcoholic fatty liver disease, obstructive sleep apnea and sleep depravation, and some forms of cancer. This cluster of maladies has been termed
"Insulin Resistance Syndrome." Therefore, an individual with insulin resistance is strongly predisposed to an increased risk
of life-threatening clinical conditions, including cardiovascular disease.
The model above depicts that with the development of insulin resistance the beta cells in the pancreas compensates by increasing the insulin response over time in years, thereby avoiding the development of measurable fasting hyperglycemia until the time that the increase in hyperglycemia (Impaired Fasting Glucose) overwhelms the insulin response or there is a decline in insulin secretion.

The clinical consequences of insulin resistance are not due to insulin resistance per se but come from the hyperinsulinemia that occurs as the individual with insulin resistance attempts to maintain normoglycemia, Meaning compensatory hyperinsulinemia. Chronic hyperinsulinemia may be beneficial to resistant tissues requiring it, for example to maintain insulin action in liver, muscle, and adipose tissues; however, it may wreak havoc with tissues that have normal sensitivity to insulin. Even within the same tissue, some of the insulin-regulated pathways, such as the glucose metabolic pathway, are more resistant to insulin than others. Therefore, intensive efforts are being directed toward identifying novel nutritional and pharmacological approaches that improve insulin sensitivity in target tissues
The Pathophysiology of insulin resistance in type 2 diabetes mellitus Insulin resistance has an important role in the pathophysiology of T2DM and CVD and both genetic and environmental factors facilitate its development. More than 90% of people with T2DM are obese, and the release of free fatty acids (FFAs) and cytokines from adipose tissue directly impairs insulin sensitivity (Figure 6). In skeletal muscle and adipose tissue, FFA-induced reactive oxygen species (ROS) production blunts activation of insulin recep tor substrate 1 (IRS-1) and PI3K-Akt signalling, leading to down regulation of insulin responsive glucose transporter 4 (GLUT-4)
Insulin resistance is a complex condition influenced by multiple factors. The following are some of the key contributors: |
---|
1. Inflammation: Chronic inflammation can interfer with insulin signaling pathways, leading to insulin resistance. Conditions like obesity and autoimmune diseases often involve inflammation. |
2. Stress: Prolonged stress increases the release of cortisol, a hormone that can promote insulin resistance by affecting glucose metabolism and increasing blood sugar levels. |
3. Insulin: Paradoxically, high levels of insulin (hyperinsulinemia) can lead to insulin resistance. This occurs when the body produces more insulin to compensate for cells not responding effectively, eventually causing the cells to become even more resistant. |
4. Urea: Elevated urea levels, often seen in kidney dysfunction, can impair insulin signaling and contribute to insulin resistance. |
5. Seed Oils: Some studies suggest that excessive consumption of certain seed oils, high in omega-6 fatty acids, may promote inflammation and insulin resistance. However, this is still a topic of ongoing research. |
6. Sleep Deprivation: Lack of sleep disrupts hormonal balance, including insulin and cortisol levels, leading to impaired glucose metabolism and increased insulin resistance. |
7. Age: As we age, the risk of insulin resistance increases due to changes in body composition, decreased physical activity, and alterations in hormone levels2. |
8. Obesity: Excess body fat, particularly around the abdomen, is a significant risk factor for insulin resistance. Fat cells release inflammatory cytokines that interfere with insulin signaling. |
9. Sedentary Lifestyle: Physical inactivity reduces the body's ability to use insulin effectively. Regular exercise helps improve insulin sensitivity. |
10. Diet: Diets high in refined carbohydrates, sugars, and unhealthy fats can contribute to insulin resistance. Conversely, a balanced diet rich in whole foods, fiber, and healthy fats can improve insulin sensitivity. |
11. Genetics: Family history and genetic predisposition play a role in the development of insulin resistance. Certain genetic variations can affect how the body responds to insulin. |
12. Hormonal Imbalances: Conditions like polycystic ovary syndrome (PCOS) and hormonal changes during menopause can increase the risk of insulin resistance. |
13. Medications: Some medications, such as corticosteroids and certain antipsychotics, can induce insulin resistance as a side effect. |

Type 2 Diabetes Mellitus (T2DM), one of the most common metabolic disorders, as shown above is caused by a combination of two primary factors:
insufficient insulin secretion by pancreatic β-cells and/or the inability of insulin-sensitive tissues to respond appropriately to insulin (insulin resistance).
Because insulin release and activity are essential processes for glucose homeostasis, the molecular mechanisms involved in the synthesis
and release of insulin, as well as in its detection are tightly regulated. Defects in any of the mechanisms involved in these processes
can lead to a metabolic imbalance responsible for the development of the disease.
Type 2 Diabetes Mellitus (T2DM) risk factors and the pathological changes leading to the perpetuation of insulin dysfunction.
Complex combinations of genetic, metabolic and environmental factors that interact with one another constitute both non-modifiable
(ethnicity and family history/genetic predisposition) and modifiable risk factors (obesity, low physical activity and an unhealthy diet).
These states affect cell function resulting in a complex network of pathological changes that influence mutually and lead to the perpetuation
of insulin dysfunction. ROS: reactive oxygen species; ER: endoplasmic reticulum; AGEs: advanced glycation end products; PKC: protein kinase C;
LPS: lipopolysaccharide; miRNA: microRNA.
This will be the objective of the following:

The interplay of the pancreas with the brain, liver, gut as well as adipose and muscle tissue. The pancreas interacts with the brain, liver, gut and adipose and muscle tissue in a highly sophisticated network via various hormones, neurotransmitters and cytokines. BNDF, brain-derived neurotrophic factor; CCK, cholecystokinin; GIP, glucose-dependent insulinotropic peptide; GLP-1, glucagon-like peptide 1; GRP, gastrin-releasing peptide; IL-6, Interleukin 6; MCH, melanin concentrating hormone; NPY, neuropeptide Y; PACAP, pituitary adenylate cyclase-activating polypeptide; POMC, pro-opiomelanocortin; VIP, vasoactive intestinal peptide.

In regards to most of the folowing informationn I am usually looking at the cell level and the biochemical pathways
that make this study so interesting. I have also included some videos on cell mechanics at the bottom.
We stay alive because millions of different biochemical reactions are taking place inside our bodies all the time.
Each of our cells is like the busy auto assembly line. Raw materials, half-finished products, and waste materials are constantly being used,
produced, transported, and excreted. The "workers" on the cellular assembly line are mainly enzymes.
These are the proteins that make biochemical reactions happen.
The sum of all the biochemical reactions in an organism is referred to as Metabolism.
Metabolism includes both exothermic (Catabolic) chemical reactions and endothermic (Anabolic) chemical reactions.
Catabolic reactions break down molecules into smaller units and release energy.
An example of a catabolic reaction is the breakdown of glucose during cellular respiration,(Glycolysis), which releases energy that cells need to carry out life
processes.
Anabolic reactions absorb energy and build bigger molecules from smaller ones.
An example of an anabolic reaction is the joining of amino acids to form a protein.( Protein Synthesis)
Metabolism is those life-sustaining chemical transformations within the cells of living organisms. The three main purposes of metabolism are: |
1. The conversion of food/fuel to energy (ATP) to run cellular processes, |
2. The conversion of food/fuel to building blocks for proteins, lipids, nucleic acids, and some carbohydrates, |
3. The elimination of Metabolic wastes. |
4. Metabolism can be impacted by age, sex, DIET, exercise, sleep, and injury or disease. |
The following are a few definitions and images of Cell processes.
A cell is the smallest living organism and the basic unit of life.
30 - 40 trillion cells make up the human body.
Cells have three parts: the membrane, the nucleus, and the cytoplasm. A typical animal cell contains more than
42,000
different kinds of molecules.
In the past 20 years, great progress has been made in understanding how these molecules combine and interact to form a living creature.
In addition to this, there are approximately
40 trillion bacteria occuping the intestinal tract that need to be fed.
Essential Characteristics of Cells explained Here
Video: Biology of Cell Structure
Nucleus:
The nucleus represents the cell's headquarters. There is typically one nucleus per cell.
However, this is not always the case. Skeletal muscle cells, for instance, have two.
The nucleus of the cell is a membrane-bound organelle found in most eukaryotic cells and is the largest organelle within the cell.
It contains nearly all of the cell's genetic material, organized into chromosomes, which are essential for gene expression and cell function.
The nuclear envelope, which surrounds the nucleus, is perforated with nuclear pores that regulate the transport of molecules between the nucleus and the cytoplasm.
(refer here for more info.)
Plasma Membrane:
To ensure each cell remains separate from its neighbor, a special membrane, known as the plasma membrane, envelops the cell.
Phospholipids make most of this membrane and prevent water-based substances from entering the cell.
Note: Cholesterol plays a distinct role in membrane structure. Cholesterol will not form a membrane by itself, but inserts into a bilayer of phospholipids.
(refer here for more info.)
GLUCOSE AND INSULIN RECEPTORS
Cells need transporters for glucose and other water-based (hydrophilic) molecules because their cell membranes are made of a lipid bilayer
that is hydrophobic (water-repelling) in nature. Imagine trying to mix oil and water—it just doesn’t work!
Glucose and other hydrophilic molecules can't pass through the lipid bilayer on their own. To get these crucial molecules into the cell,
specialized transport proteins act as gateways. These transporters provide a way for glucose and other water-soluble molecules to cross the
cell membrane without getting stuck. It’s a bit like having a key to unlock the door and let in what the cell needs to function and thrive.
The plasma membrane contains a range of receptors, which carry out a number of tasks, including being: |
---|
1. Gatekeepers: Some receptors allow certain molecules through and stop others. |
2. Markers: These receptors act as name badges, informing the immune system that they are part of the organism and not foreign invaders. |
3. Communicators: Some receptors help the cell communicate with other cells and the environment. |
4. Fasteners: Some receptors help bind the cell to its neighbors. |
In total, there are hundreds of different types of receptors, each playing a crucial role in cellular communication and function. |
GLUCOSE RECEPTORS
At this point in the discussion as we are concerned with insulin and insulin resistance and the two main receptors are the insulin and glucose receptors.
There are approimately 14 glucose receptors and of those there are 5 of major concern.
An insulin-dependent glucose transporter, GLUT4, plays a crucial role in glucose regulation in Skeletal muscle, adipose cells (fat cells), the heart
and some brain cells.
Muscle cells are significant consumers of glucose in the body. Approximately 80% of the glucose from food is processed by muscle cells.
This high percentage underscores the importance of muscle tissue in maintaining overall metabolic health and regulating blood glucose levels.
GLUT4 is responsible for the facilitated diffusion of glucose into these cells. It is unique because its activity is regulated by insulin,
making it essential for maintaining normal blood glucose levels.
Refer to Source here
Schematic of insulin-induced translocation of glucose transporter 4 from cytosol to the cell membrane.
The binding of insulin to its receptors initiates a signal transduction cascade, which results in the activation of Akt.
Akt acts on the glucose transporter 4 (GLUT4) containing vesicles in the cytosol to facilitate their fusion with the cell membrane.
When more GLUT4 molecules are present in the membrane, the rate of glucose uptake is elevated. GLUT4: Glucose transporter 4.

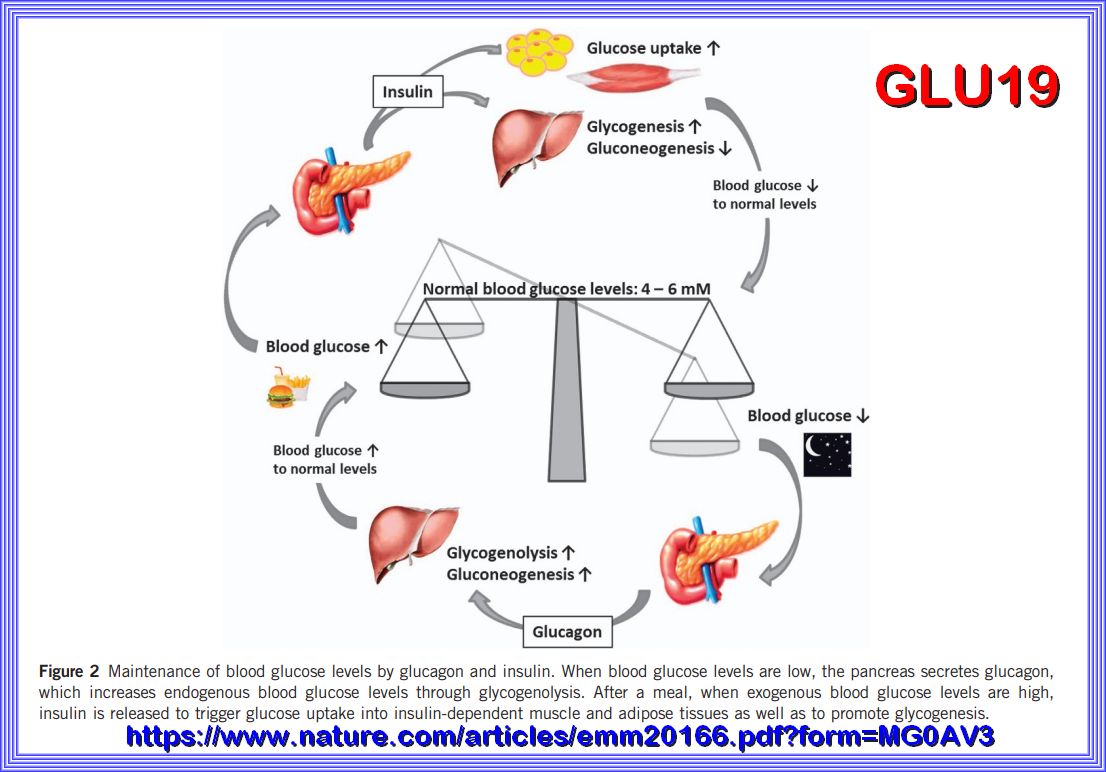
Refer here for siurce information.
In order to ensure normal body function, the human body is dependent on a tight control of its blood glucose levels. Note the 4 to 6 mM is a
Europe designation, that would approximate 70 to 100 mg/l in the us system as what would be normal fasting blood glucose levels. This arbitrary as the fasting level
should be closer to the 80 range. a fasting Glucose level of 100 to 126 is considered pre diabetic and over 126 is Diabetic This balance is accomplished
by a highly sophisticated network of various hormones and neuropeptides released mainly from the brain, pancreas, liver, intestine as well
as adipose and muscle tissue. Within this network, the pancreas represents a key player by secreting the blood sugar-lowering hormone insulin
and its opponent glucagon. However, disturbances in the interplay of the hormones and peptides involved may lead to metabolic disorders
such as type 2 diabetes mellitus (T2DM) whose prevalence, comorbidities and medical costs take on a dramatic scale.
GLU19 above shows the Maintenance of blood glucose levels by glucagon and insulin. When blood glucose levels are low, the pancreas secretes glucagon,
which increases endogenous blood glucose levels through glycogenolysis. After a meal, when exogenous blood glucose levels are high,
insulin is released to trigger glucose uptake into insulin-dependent muscle and adipose tissues as well as to promote glycogenesis.
MECHANISM: |
---|
1. Insulin Binding: When blood glucose levels rise, insulin is released from the pancreas. |
2. Signal Transduction: Insulin binds to its receptor on the cell surface, initiating a signaling cascade. |
3. GLUT4 Translocation: This signaling causes GLUT4-containing vesicles within the cell to move to the plasma membrane. |
4. Glucose Uptake: GLUT4 integrates into the membrane, allowing glucose to enter the cell from the bloodstream. |
Importance |
5. Energy Storage: In muscle cells, glucose is used for energy production or stored as glycogen. |
6. Fat Storage: In adipose tissue, glucose is converted into fat for long-term energy storage. |
7. Amino acids are converted to protein for muscle hypertrophy. |
REGULATION: |
8. Exercise: Physical activity can also stimulate GLUT4 translocation to the cell membrane, independent of insulin. |
9. Insulin Resistance: In conditions like Type 2 Diabetes, the efficiency of GLUT4 translocation is impaired, leading to elevated blood glucose levels. |
Understanding GLUT4's role helps in grasping how the body manages glucose and the impact of insulin resistance on metabolic health. |
INSULIN RECEPTOR
This animation describes the role of the insulin receptor in type 2 diabetes. It focuses on the very recent discovery of
how the hormone insulin actually binds to the receptor on the surface of cells, as determined by Professor Mike Lawrence's laboratory at
the Walter and Eliza Hall Institute.
Insulin binds to the receptor protein on the cell surface and instructs the cell to take up glucose from the blood for use as an energy source.
In type 2 diabetes, they believe that insulin binds to the receptor normally, but the signal is not sent into the cell, the cells do not take up
glucose and the resulting high blood glucose levels cause organ damage over time.
PANCREAS
The pancreatic β-cell plays a key role in glucose homeostasis by secreting insulin, the only hormone capable of lowering the blood glucose concentration.
Impaired insulin secretion results in the chronic hyperglycemia that characterizes type 2 diabetes (T2DM).
The healthy β-cell acts as a glucose sensor matching its output to the circulating glucose concentration.
It does so via metabolically induced changes in electrical activity, which culminate in an increase in the cytoplasmic Ca2+ concentration and initiation
of Ca2+-dependent exocytosis of insulin-containing secretory granules.
CYTOPLASM
The cytoplasm is the interior of the cell that surrounds the nucleus. It includes the organelles and a jelly-like fluid called the cytosol.
Many of the important reactions that take place in the cell occur in the cytoplasm such as:
1. Glycolysis: This is the first stage of cellular respiration, where glucose is broken down into pyruvate, producing a small amount of
ATP and NADH.
2. Protein Synthesis: The translation of mRNA into proteins occurs on ribosomes, which are often found in the cytoplasm.
3. Cell Division: Processes such as mitosis and meiosis, which are essential for cell replication and reproduction, begin in the cytoplasm.
3. Metabolic Pathways: Various metabolic pathways, including those involved in the synthesis and breakdown of molecules, occur in the cytoplasm.
These reactions are crucial for the cell's energy production, growth, and overall function and are discussed below.
Mitochondria:
The mitochondrion is the organelle of a cell that is actively involved in the production of energy or fuel (ATP) for the
basic biological functioning of a cell. ATP - or adenosine triphosphate - is a high-energy compound that
fuels cellular energy requirements.
It is produced by the process of cellular respiration that takes place in the mitochondrion.
During cellular respiration the food is oxidized, oxygen is consumed, and carbon dioxide is released.
Cellular respiration is the metabolic process that occurs in the cells of living organisms.
It involves converting chemical energy from nutrients, such as glucose, into adenosine triphosphate (ATP),
which cells use for energy.
This process also produces carbon dioxide and water as waste products.
The main stages of cellular respiration include:
IN THE CYTOPLASM:
Glycolysis: The breakdown of glucose into pyruvate, producing a small amount of ATP and NADH.
IN THE MITOCHONDRIA:
Citric Acid Cycle (Krebs Cycle, TCA Cycle): The pyruvate is further broken down, generating more NADH and FADH2, and releasing carbon dioxide.
Oxidative Phosphorylation (OXPHOS):
The NADH and FADH2 produced in the previous stages donate electrons to the electron transport chain, driving the production of a large amount of
ATP.
This process is essential for providing the energy required for various cellular activities and maintaining life.
The ATP-ADP cycle:
the ATP-ADP cycle is an essential process in cellular energy metabolism.
ATP (adenosine triphosphate) is the energy-carrying molecule in cells.
ATP releases energy when one of its three phosphate molecules breaks free,(Phosphorylation)
becoming ADP (adenosine diphosphate).
ADP can be recycled back into ATP by adding a phosphate molecule.
ATP is useful in many cell processes such as glycolysis, photosynthesis, beta oxidation, anaerobic respiration,
active transport across cell membranes (as in the electron transport chain), and synthesis of macromolecules such as DNA.
Phosphorylation is an important process as the chemical addition of a phosphoryl group (PO3-) to an organic molecule.
This process involves the transfer of a phosphate group from a donor molecule, typically ATP (adenosine triphosphate),
to an acceptor molecule, such as a protein or small molecule.
1. Phosphorylation is a biochemical process that involves the addition of a phosphate group via an ester bond, usually catalyzed by enzymes called
kinases.
2. It can occur on proteins, small molecules, or carbohydrates, leading to changes in their structure, function, or activity.
3. Phosphorylation is a reversible process, as enzymes called phosphatases can remove phosphate groups
(dephosphorylation) from molecules.
An enzyme is a protein that speeds up a biochemical reactions. It is a biological catalyst. An enzyme generally works by reducing the amount of activation energy needed to start the reaction. the activation energy needed for glucose to combine with oxygen to produce carbon dioxide and water. The overall reaction releases energy, but an initial activation energy is needed to start the process. The activation energy without an enzyme is much higher than the activation energy when an enzyme is used.
Key Metabolic Process Definitions
1. Gluconeogenesis: Synthesis of glucose using non-carbohydrate precursors. |
2. Glycolysis: Degradation of glucose into pyruvic acid and energy for cell metabolism. |
3. Glycogenesis: Synthesis of glycogen from glucose. |
4. Glycogenolysis: Degradation of glycogen into glucose. |
5. Lipogenesis: Conversion of acetyl-CoA into fatty acids and subsequent triglyceride synthesis. |
6. Lipolysis: Degradation of lipids and triglycerides into free fatty acids. |
7. Protein synthesis: The biological process by which amino acids are assembled into specific polypeptides. |
8. Apoptosis: A genetically directed process of cell self-destruction that is marked by the fragmentation of nuclear DNA. |
Citric Acid Cycle (2020) by Drew Berry wehi.tv
Pyruvate Dehydrogenase Complex (2021) Drew Berry wehi.
Electron Transport Chain (2019) Drew Berry wehi.
Electron Transport Chain (2019) Drew Berry wehi.
Pathophysiology of Type 2 Diabetes Mellitus
Regulatory principles in metabolism -Then and now
Diagnosis and Management of the Metabolic Syndrome
Cellular mechanisms of insulin resistance
Sympathetic nervous activation in metabolic syndrome and obesity
Insulin Resistance and Hyperinsulinemia
Metabolic syndrome: a sympathetic disease?
Saturated Fat with Dr. Ben Bikman
Insulin Resistance and Metabolic Syndrome with Dr. Ben Bikman
Albuminuria: An Underappreciated Risk Factor for Cardiovascular Disease
Enzymes: principles and biotechnological applications
Non-insulin dependent diabetes mellitus: the gathering storm
Social, clinical, and policy implications of ultra processed food addiction
Regulation of Cellular Respiration
The Disparate Effects of Epinephrine and Norepinephrine on Hyperglycemia in Cardiovascular Surgery
Association between triglyceride glucose index and biological aging in U.S. adults:
Defining and characterizing the progression of type 2 diabetes
ESC Guidelines on diabetes, pre-diabetes, and cardiovascular diseases
Improvement in Glycemic and Lipid Profiles in Type 2 Diabetics with a 90-Day Ketogenic Diet
CD38 ecto-enzyme in immune cells is induced during aging regulating NAD+ and NMN levels
NAD+ metabolism: pathophysiologic mechanisms and therapeutic potential
GLYCOLYSIS
Glycolysis represents the process of breaking down monosaccharides, which involves the energy metabolism, homeostasis,
and the linkage of various physiological functions such as muscle movement, development, neurotransmission, etc.
what happens when there are Elevated levels of ATP and alanine create negative feedback to inhibit PK, does the flow reverese bak to g6p
When there are elevated levels of ATP and alanine, they act as negative feedback inhibitors for pyruvate kinase (PK). This inhibition slows down the conversion of phosphoenolpyruvate (PEP) to pyruvate in glycolysis. As a result, the flow of metabolites is redirected upstream.
However, the flow does not reverse back to glucose-6-phosphate (G6P). Instead, the accumulation of PEP and other intermediates can
lead to an increase in the levels of fructose-1,6-bisphosphate (F1,6BP), which can then be converted back to fructose-6-phosphate (F6P)
and eventually to G6P through gluconeogenesis or other pathways
Phosphofructokinase is the main enzyme controlled in glycolysis. High levels of ATP, citrate, or a lower, more acidic pH decrease the
enzyme’s activity. An increase in citrate concentration can occur because of a blockage in the citric acid cycle. Fermentation, with
its production of organic acids like lactic acid, frequently accounts for the increased acidity in a cell; however, the products of fermentation
do not typically accumulate in cells.
Glycolysis: A multifaceted metabolic pathway and signaling hub
Glycolysis: A multifaceted metabolic pathway and signaling hub
Alcohol and Metabolic-associated Fatty Liver Disease
Aging and Insulin Resistance: Just Say iNOS
Regulation of Glycolysis: Short and Long-Term Control Mechanisms
AGING
Metabolite and protein associations with general health in the population
Aging and Insulin Resistance: Just Say iNOS
Insulin Resistance Accelerates Biological Aging as Measured by Aging Clocks
IFG-IGT
IFG-IGT
Diagnosis, Prognosis, and Treatment of Impaired Glucose Tolerance and Impaired Fasting Glucose
VIDEO Understanding Type 2 Diabetes
STATISTA: What share of adults are obese
Insulin Resistance: From Mechanisms to Therapeutic Strategies
Insulin resistance - Reference pathway
METABOLIC SYNDROME
(FKA INSULIN RESISTANCE SYNDROME)
Metabolic syndrome represents the clinical diagnostic entity identifying those individuals at high risk with respect to the
(cardiovascular) morbidity associated with insulin resistance
The National Cholesterol Education Program and other organizations have proposed that the MetS can be recognized clinically by a clustering
of simple clinical measures including waist circumferences, blood pressure, triglycerides, high-density lipoproteins, and glucose.
People with this clustering have most or all of the components of the MetS.
Identifying the MetS has several advantages. It discovers persons who are at increased risk for cardiovascular disease.
Increased age and metabolic syndrome are the most important relevant factors for diabetes mellitus,
especially by using the International Diabetes Federation criteria for definition of the metabolic syndrome.
The Global Epidemic of the Metabolic Syndrome
Pathophysiology of Type 2 Diabetes Mellitus
The impact of diabetes on cognitive impairment and its progression to dementia
Overview of Metabolic Reactions
Chemical Reactions in Living Things
Amino Acid Ingestion Strongly Enhances Insulin Secretion in Patients With Long-Term Type 2 Diabetes
Reassessment of Glucose Effectiveness and Insulin Sensitivity From Minimal Model Analysis
Diagnosis and Management of the Metabolic Syndrome
Lipid-Overloaded Enlarged Adipocytes Provoke Insulin Resistance Independent of Inflammation
Metabolic Inflexibility: When Mitochondrial Indecision Leads to Metabolic Gridlock
Metabolic Flexibility as an Adaptation to Energy Resources and Requirements in Health and Disease
Metabolic flexibility and insulin resistance
The Carbohydrate-Insulin Model of Obesity: Beyond ‘Calories In, Calories Out’
How monitoring ketones and glucose can help you achieve metabolic flexibility
Diagnosis and Management of the Metabolic Syndrome
Metabolic syndrome – a new definition and management guidelines
Non-insulin dependent diabetes mellitus: the gathering storm
Metabolic Inflexibility: When Mitochondrial Indecision Leads to Metabolic Gridlock
Mechanisms of Insulin Action and Insulin Resistance
What’s on your table? How America’s diet has changed over the decades
Effect of circadian clock disruption on type 2 diabetes
Metabolism Disrupting Chemicals and Metabolic Disorders
Glucose control of glucagon secretion
The Central Role of Glucokinase in Glucose Homeostasis
The somatostatin-secreting pancreatic δ-cell in health and disease
Monitoring and modelling the dynamics of the cellular glycolysis pathway
The Central Role of Glucokinase in Glucose Homeostasis
Metabolic Effects of Late Dinner in Healthy Volunteers
Video: What if Heart Disease and Diabetes had the same cause? | Ivor Cummins
The Metabolic Interplay between Cancer and Other Diseases
Molecular Mechanisms of Glucocorticoid-Induced Insulin Resistance
Molecular Mechanisms of Glucocorticoid-Induced Insulin Resistance
Assessing Insulin Sensitivity and Resistance in Humans
Circadian phase inversion causes insulin resistance in a rat model of night work and jet lag
Trapped fat: Obesity pathogenesis as an intrinsic disorder in metabolic fuel partitioning
Nonlinear dynamics of multi-omics profiles during human aging
Metabolic Syndrome, is a cluster of comorbid conditions including obesity, hypertension, and disordered carbohydrate and lipid metabolism,
constitutes a significant health and social problems, which occur together more often than by chance alone:
Although obesity is a well-known risk factor for poor Metabolic Health, Metabolic Health issues such as insulin resistance and diabetes
risk also affect normal-weight people. A useful method for assessing Metabolic health is to determine the presence of Metabolic Syndrome,
which is defined as having 3 of the following 5 criteria: central obesity, elevated blood glucose, elevated triglycerides, low levels
of high-density lipoprotein cholesterol, and elevated blood pressure.
Other than genetics, Metabolic Syndrome is highly correlated with unhealthy diet, unhealthy sleeping patterns, sedentary behaviors, and physical inactivity.
Elevated waist circumference ≥ 40 inches in men; |
Elevated waist circumference ≥ 35 inches in women; |
Elevated triglycerides ≥150 mg/dL (1.7 mmol/L); |
Reduced HDL-C ≤40 mg/dL (1.0 mmol/L) in males; |
Reduced HDL-C ≤50 mg/dL (1.3 mmol/L) in females; |
Elevated blood pressure Systolic ≥130 and/or diastolic ≥85 mm Hg; |
Elevated fasting glucose ≥100 mg; |
Note Some US adults of non-Asian origin (eg, white, black, Hispanic) with marginally increased waist circumference
(eg,[37–39 inches] in men and [31–34 inches] in women) may have strong genetic contribution to
insulin resistance and should benefit from changes in lifestyle habits, similar to men with categorical increases in waist circumference.
Lower waist circumference cut point (eg,[35 inches] in men and (31 inches) in women) appears to be appropriate for Asian Americans.
Aso, certain drug treatments or Docter suppervised programs may alter the above guidelines.
Glycated haemoglobin: 2023 ESC Guidelines
Following high-quality epidemiological studies, it was suggested that HbA1c could be used to diagnose diabetes, and this was subsequently
endorsed by international guidelines. It should be noted that epidemiological studies have relied on the adult population, though HbA1c
is also used in younger individuals as a diagnostic test. Advantages of HbA1c include ease of measurement, limited within-individual
variability, and the convenience of anytime testing without the need for fasting or a cumbersome OGTT.
However, HbA1c is not accurate in specific groups where the relationship between HbA1c and glucose levels is altered for any reason
Moreover, in cases of shorter diabetes duration, such as early type 1 diabetes mellitus
(T1DM) or acute pancreatic damage, HbA1c can lead to false-negative results. Another practical limitation is the lack of test availability
in some parts of the world due to financial constraints.
Guidelines agree that HbA1c ≥48 mmol/mol (≥6.5%) is diagnostic of diabetes, while the diagnosis of pre-diabetes uses two different
cut-off values. The WHO criteria define pre-diabetes as HbA1c 42–47 mmol/mol (6.0–6.4%), while the ADA recommends a wider range of
39–47 mmol/mol (5.7–6.4%). Notably, the combination of HbA1c and fasting glucose in the diabetes range is diagnostic of diabetes
and a second test is not required, even if the individual is asymptomatic. However, if the two are discordant, the number in the
diabetes range should be repeated or, preferably, an OGTT performed, which remains the gold standard for diagnosing diabetes in
unclear cases. The criteria used for diagnosing diabetes and pre-diabetes are summarized in Table 6. It should be noted that data
from 73 studies on 294 998 individuals without known diabetes suggest that HbA1c is as good as or better than fasting, random, or
post-load glucose levels for predicting CV risk. Refer to the ESC 2023 guidelines below.
Diagnostic Criteria for Metabolic Syndrome
VIDEO: The American Diabetes Association’s Standards of Care in Diabetes—2024
If you Would you like to run a Metabolic Health Anaysis based on the Metabolic Syndrome with some information from your current lipid panel use this:
INSULIN, GLUCAGON & HYPERINSULINEMIA
Insulin is a peptide hormone secreted by the β cells of the pancreatic islets of Langerhans and maintains normal blood glucose levels
by facilitating cellular glucose uptake, regulating carbohydrate, lipid and protein metabolism and promoting cell division and growth through its mitogenic effects.
Insulin resistance is defined where a normal or elevated insulin level produces an attenuated biological response; classically this refers
to impaired sensitivity to insulin mediated glucose disposal.
Compensatory hyperinsulinaemia occurs when pancreatic β cell secretion increases to maintain normal blood glucose levels in the setting of
peripheral insulin resistance in muscle and adipose tissue.
In most natural habitats, calorie availability is scarce and unpredictable, necessitating the evolution of systems for the efficient storage and
utilization of energy. But in our modern, mechanized society, caloric demands are minimized, while highly palatable, calorie-dense foods and
beverages are readily available. These changes have fostered the current pandemic of obesity and comorbid conditions of nonalcoholic fatty liver
disease (NAFLD), atherosclerosis, and type 2 diabetes (T2D).
Here is a possible representation of the relationship between hyperinsulinemia and fasting hyperglycemia:
Years 1-5: Insulin resistance increases, leading to hyperinsulinemia (≥25 mIU/ml) with normal Glucose levels.
Years 5-10: Hyperinsulinemia persists, and insulin resistance worsens, leading to impaired glucose tolerance and prediabetes:
( 100-125mg/dL or 5.6-6.9 mmol/L)
Years 10-15: Fasting hyperglycemia (≥126 mg/dL or 6.9 mmol/L) develops, indicating the onset of Type 2 diabetes
Insulin maintains normal glucose levels despite increasing insulin resistance through a process called compensatory hyperinsulinemia.
Increased Insulin Secretion: As insulin resistance develops, the body’s cells (particularly muscle, fat, and liver cells) become less responsive
to insulin. To compensate for this reduced sensitivity, the pancreas produces and secretes more insulin. This increased insulin production helps to
maintain normal blood glucose levels by ensuring that glucose can still be taken up by cells for energy or storage
Enhanced Beta Cell Function: The pancreatic beta cells, which are responsible for producing insulin, work harder and become more efficient
at secreting insulin in response to rising blood glucose levels. This enhanced function helps to counteract the effects of insulin resistance
Suppression of Hepatic Glucose Production: Insulin plays a crucial role in suppressing the liver’s production of glucose.
With higher levels of insulin, the liver’s glucose output is reduced, which helps to keep blood glucose levels within the normal range.
Increased Insulin Sensitivity in Other Tissues: While insulin resistance primarily affects muscle, fat, and liver cells,
other tissues in the body may remain more sensitive to insulin. This differential sensitivity helps to maintain overall glucose homeostasis.
Feedback Mechanisms: The body has several feedback mechanisms that help regulate insulin and glucose levels. For example, when blood glucose levels rise,
the pancreas releases more insulin. Conversely, when blood glucose levels fall, insulin secretion decreases. These feedback loops help to maintain
glucose levels within a narrow range.
Despite these compensatory mechanisms, over time, the pancreas may become unable to produce enough insulin to overcome the increasing resistance.
When this happens, blood glucose levels start to rise, leading to conditions such as Impaired Fasting Glucose (IFG) and eventually type 2 diabetes.
Role of PI3K/AKT Pathway in Insulin-Mediated Glucose Uptake
The pathogenesis of insulin resistance: integrating signaling pathways and substrate flux
Prediabetes: A high-risk state for developing diabetes
Hyperinsulinemia: an early biomarker of metabolic dysfunction
Insulin Resistance and Hyperinsulinemia
Hyperinsulinemia in Obesity, Inflammation, and Cancer
Hyperglycaemia reduces gastrin-stimulated gastric acid secretion in humans
β Cell GHS-R Regulates Insulin Secretion and Sensitivity
Hepatic Insulin Clearance: Mechanism and Physiology
Hyperglycemia alters PI3k and Akt signaling and leads to endothelial cell proliferative dysfunction
Hyperinsulinemia: An Early Indicator of Metabolic Dysfunction
Molecular Physiology of Insulin Function
Insulin and Insulin Resistance
Mechanisms of Insulin Action and Insulin Resistance
The Insulin Receptor and Its Signal Transduction Network
Activation mechanism of the insulin receptor: a structural perspective
The visceral adiposity index is a predictor of incident nonalcoholic fatty liver disease:
Changes in Cells Associated with Insulin Resistance
Targeting hepatic glucose output in the treatment of type 2 diabetes
Pathogenesis of Insulin Resistance in Skeletal Muscle
The pathogenesis of insulin resistance: integrating signaling pathways and substrate flux
Insulin Signaling And Insulin Resistance
Lipid-induced insulin resistance: unravelling the mechanism
Ectopic Fat and Insulin Resistance: Pathophysiology and Effect of Diet and Lifestyle Interventions
Insulin Degradation: Progress and Potential*
Role of Insulin Clearance in Insulin Action and Metabolic Diseases
The Physiology of Insulin Clearance
Insulin Resistance and Hyperinsulinemia: Is hyperinsulinemia the cart or the horse?
Cephalic phase insulin release: A review of its mechanistic basis and variability in humans
Glucose‐stimulated insulin secretion: A newer perspective
The Hidden Problem of Chronic Hyperinsulinemia
Mechanisms of muscle insulin resistance and the cross-talk with liver and adipose tissue
Recent Advances in Our Understanding of Insulin Action and Insulin Resistance
Insulin–Heart Axis: Bridging Physiology to Insulin Resistance
Insulin signalling and the regulation of glucose and lipid metabolism
Insulin action and resistance in obesity and type 2 diabetes
Diabetes: Have We Got It All Wrong?
Prediabetes: A high-risk state for developing diabetes
Insulin Resistance Mayo Clinic
Hyperinsulinemia: An Early Indicator of Metabolic Dysfunction
Current Studies on Molecular Mechanisms of Insulin Resistance
Insulin: too much of a good thing is bad
The pathogenesis of insulin resistance: integrating signaling pathways and substrate flux
Mechanisms of Insulin Action and Insulin Resistance
Defining and Characterizing the Progression of Type 2 Diabetes
Mechanisms of β-Cell Death in Type 2 Diabetes
The role of interleukin-1β in type 2 diabetes mellitus: A systematic review and meta-analysis
Insulin and aging – a disappointing relationship
Probing the Relationship Between Insulin Sensitivity and Longevity Using Genetically Modified Mice
Insulin Resistance: The Increased Risk of Cancers
Plant-Based Diet Indices and Their Association with Frailty in Older Adults
Endocrinology of the Aging Prostate: Current Concepts
https://pmc.ncbi.nlm.nih.gov/articles/PMC9135930/
Dopamine Negatively Regulates Insulin Secretion
Insulin resistance in brain alters dopamine turnover and causes behavioral disorders
Insulin Regulates Brain Function, but How Does It Get There
Does Insulin Play a Role in Prostate Cancer
Fasting Insulin and Risk of Cancer Related Mortality in Non-diabetic Adults
A review of the carbohydrate–insulin model of obesity
The Carbohydrate-Insulin Model of Obesity: Beyond "Calories In, Calories Out"
Relationship Between Insulin Resistance and an Endogenous Nitric Oxide Synthase Inhibitor
Hyperinsulinemia in Obesity, Inflammation, and Cancer
Diabetes: Have We Got It All Wrong?
Regulation of insulin secretion: a matter of phase control and amplitude modulation
Paracrine regulation of insulin secretion
Abnormal pancreatic glucagon secretion and postprandial hyperglycemia in diabetes mellitus
The Difference δ-Cells Make in Glucose Control
INSULIN RECEPTOR
Insulin Receptor Trafficking: Consequences for Insulin Sensitivity and Diabetes
Insulin Receptor Signaling in Normal and Insulin-Resistant States
The role of GLUT2 in glucose metabolism in multiple organs and tissues
The facilitative glucose transporter GLUT3: 20 years of distinction
Glucose transporters in pancreatic islets
Hypertonicity during a rapid rise in D-glucose mediates first-phase insulin secretion
Glucose transporters in pancreatic islets
Current understanding of glucose transporter 4 expression and functional mechanisms
Regulation of insulin receptor function
The Insulin Receptor and Its Signal Transduction Network
Insulin Receptor Signaling in Normal and Insulin-Resistant States
Agonism and Antagonism at the Insulin Receptor
GLUCOSE & HYPERGLYCEMIA
The term "Hyperglycemia" is derived from the Greek hyper (high) + glykys (sweet/sugar) + haima (blood).
Hyperglycemia is defined as blood glucose greater than 125 mg/dL while fasting and greater than 180 mg/dL 2 hours postprandial.
A patient has impaired glucose tolerance, or pre-diabetes, with a fasting plasma glucose of 100 mg/dL to 125 mg/dL.
Chronic exposure to a glucose rich environment creates several physiological and pathophysiological changes.
There are several pathways by which hyperglycemia exacerbate its toxic effect on cells, tissues and organ systems.
Hyperglycemia can induce oxidative stress, upsurge polyol pathway, activate protein kinase C (PKC), enhance
hexosamine biosynthetic pathway (HBP), promote the formation of advanced glycation end-products (AGEs) and finally
alters gene expressions. Prolonged hyperglycemic condition leads to severe diabetic condition by damaging the
pancreatic β cell and inducing insulin resistance. I hope to cover most of these concerns with the appropriate
studies and papers, some published and some in process. The idea is to try and understand what hyperglycemia and hyperinsuinemia
do to the different pathways and body organs. I have found that this is a task beyond my comprehension, but there are
studies that I can relate to and can help others to appreciate.
- Pathogenesis of Chronic Hyperglycemia: From Reductive Stress to Oxidative Stress
Prediabetes: A high-risk state for developing diabetes
ADA: Hyperglycemia (High Blood Glucose)
Vascular Dysfunction in Hyperglycemia
ABSTRACT: Hyperglycemia, lipoprotein glycation, and vascular disease
VIDEO: The Effects of Hyperglycemia on the Immune System
Diabetes and mitochondrial function: Role of hyperglycemia and oxidative stress
Circadian Regulation of Glucose, Lipid, and Energy Metabolism in Humans
Regulation of glucose metabolism from a liver-centric perspective
Impact of HbA1c Testing at Point of Care on Diabetes Management
A Practical Review of C-Peptide Testing in Diabetes
The Role of CD36 in Type 2 Diabetes Mellitus: β-Cell Dysfunction and Beyond
Effect of hyperglycemia on gastric acid secretion during the gastric phase of digestion
The Relationship between Erythrocytes and Diabetes Mellitus
Effect of high glucose concentrations on human erythrocytes in vitro
Type 2 Diabetes Mellitus: A Pathophysiologic Perspective
Updates in the Management of Hyperglycemic Crisis
Relationships BetweenGastric Emptying, Postprandial Glycemia,and Incretin Hormone
Postprandial Hyperlipidemia: Its Pathophysiology, Diagnosis, Atherogenesis, and Treatments
Effect of acute hypohydration on glycemic regulation in healthy adults
The Effect of Short-Term Hyperglycemia on the Innate Immune System
Characteristics of glucose transporters
Role of insulin and other related hormones in energy metabolism
INSULIN, MUSLE UPTAKE, AND HEXOKINASE
VIDEO: The Effects of Hyperglycemia on the Immune System
VIDEO: How diabetes destroys the human body
VIDEO: Hyperglycemia and neuropathy, nephropathy and retinopathy. DM complications
Potential Role of Protein Kinase C in the Pathophysiology of Diabetes-Associated Atherosclerosis
Impaired glucose tolerance and impaired fasting glucose share similar underlying pathophysiologies
The Promising Frontier of Cardiometabolic Syndrome: A New Paradigm in Cardiology
p38 Mitogen-activated Protein Kinase Plays a Stimulatory Role in Hepatic Gluconeogenesis
The Aging Vasculature: Glucose Tolerance, Hypoglycemia and the Role of the Serum Response Factor
Age-related Changes in Glucose Metabolism, Hyperglycemia, and Cardiovascular Risk
Metabolic flux and the regulation of mammalian cell growth
Pancreatic regulation of glucose homeostasis
Insulin, Muscle Glucose Uptake, and Hexokinase:
Regulation of GLUT4 and Insulin-Dependent Glucose Flux
Repeated glucose spikes and insulin resistance synergistically deteriorate endothelial function
GLYCOLYSIS
Glycolysis represents the process of breaking down monosaccharides, which involves the energy metabolism, homeostasis,
and the linkage of various physiological functions such as muscle movement, development, neurotransmission, etc.
what happens when there are Elevated levels of ATP and alanine create negative feedback to inhibit PK, does the flow reverese bak to g6p
When there are elevated levels of ATP and alanine, they act as negative feedback inhibitors for pyruvate kinase (PK). This inhibition slows down the conversion of phosphoenolpyruvate (PEP) to pyruvate in glycolysis. As a result, the flow of metabolites is redirected upstream.
However, the flow does not reverse back to glucose-6-phosphate (G6P). Instead, the accumulation of PEP and other intermediates can
lead to an increase in the levels of fructose-1,6-bisphosphate (F1,6BP), which can then be converted back to fructose-6-phosphate (F6P)
and eventually to G6P through gluconeogenesis or other pathways
Phosphofructokinase is the main enzyme controlled in glycolysis. High levels of ATP, citrate, or a lower, more acidic pH decrease the
enzyme’s activity. An increase in citrate concentration can occur because of a blockage in the citric acid cycle. Fermentation, with
its production of organic acids like lactic acid, frequently accounts for the increased acidity in a cell; however, the products of fermentation
do not typically accumulate in cells.
GLYCONEOGENESIS
Glycolysis and Gluconeogenesis - Reciprocal Regulation
The neglected PCK1/glucagon (inter)action in nutrient homeostasis beyond gluconeogenesis
Diabetic-induced alterations in hepatic glucose and lipid metabolism:
GLYCOGENOLESIS
Glucagon is a hormone produced by the alpha cells of the pancreas and plays a key role in regulating blood sugar levels.
Glucagon acts in opposition to insulin: while insulin promotes glucose uptake and storage, glucagon promotes the breakdown of glycogen
stores in the liver and the release of glucose into the bloodstream.
The switch from insulin secretion to glucagon secretion typically occurs when blood glucose levels start to decrease. The transition between insulin
and glucagon secretion is tightly regulated to maintain blood sugar balance.
In general, glucagon secretion is stimulated when blood glucose levels drop below a certain threshold. The approximate threshold for glucagon
secretion is around 70-80 mg/dL (3.9-4.4 mmol/L) of blood glucose. When blood glucose levels decrease below this threshold, the pancreas
responds by reducing insulin secretion and increasing glucagon secretion to promote the release of glucose from glycogen stores in the liver.
During periods of fasting, prolonged exercise, or low-carbohydrate intake, blood glucose levels can decrease, triggering glucagon
secretion to maintain blood sugar levels and provide energy for the body's cells.
Overall, the switch from insulin secretion to glucagon secretion occurs in response to decreasing blood glucose levels and is an
essential mechanism for maintaining blood sugar balance and supporting energy needs during fasting or periods of increased demand.
Glucokinase intrinsically regulates glucose sensing and glucagon secretion in pancreatic alpha cells
Glucose Controls Glucagon Secretion by Regulating Fatty Acid Oxidation in Pancreatic α-Cells
The Regulation and Secretion of Glucagon in Response to Nutrient Composition
Role of GLUT1 in regulation of reactive oxygen species
Glucagon Receptor Signaling and Glucagon Resistance
The Vicious Circle of Hepatic Glucagon Resistance in Non-Alcoholic Fatty Liver Disease
Glucagon Receptor Signaling and Glucagon Resistance
GLYCONEOGENOLYSIS
GLUT TRANSPORTERS
Glucose transporters: physiological and pathological roles
Glucose transporter 1 in health and disease
Functional Properties and Genomics of Glucose Transporters
INSULIN, MUSLE UPTAKE, AND HEXOKINASE
Glucose transport and sensing in the maintenance of glucose homeostasis and metabolic harmony
Glucose transporters in pancreatic islets
The role of GLUT2 in glucose metabolism in multiple organs and tissues
The facilitative glucose transporter GLUT3: 20 years of distinction
Glucose transporters in pancreatic islets
Glucose transporters in pancreatic islets
Current understanding of glucose transporter 4 expression and functional mechanisms
Importance of GLUT Transporters in Disease Diagnosis and Treatment
GLUT5: structure, functions, diseases and potential applications
The role of GLUT2 in glucose metabolism in multiple organs and tissues
ABSTRACT ONLY: Regulation of glucose transport by insulin: traffic control of GLUT4
GLUT2, glucose sensing and glucose homeostasis
Glucose transporters in adipose tissue, liver, and skeletal muscle in metabolic health and disease
Metabolic changes in aging humans
Alternative routes to the cell surface underpin insulin-regulated membrane trafficking of GLUT4
Insulin signalling and GLUT4 trafficking in insulin resistance
Glucose transporters in adipose tissue, liver, and skeletal muscle in metabolic health and disease
Diet & DIGESTION
OPEN
Effect of hyperglycemia on gastric acid secretion during the gastric phase of digestion
Alcohol and gastric acid secretion in humans.
Neuroendocrine control of appetite and metabolism
Autonomic control of energy balance and glucose homeostasis
Fat Digestion - Lipolysis & Lipid Transport DM
Omega-6 vegetable oils as a driver of coronary heart disease: the oxidized linoleic acid hypothesis
Insulin Resistance of Protein Metabolism in Type 2 Diabetes and Impact on Dietary Needs:
Vitamins Important for Metabolism
OVERNUTRITION
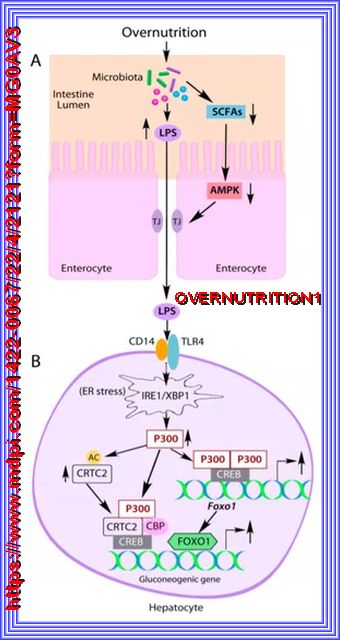
A high-fat, Western-style diet is an important predisposing factor for the onset of type 2 diabetes and obesity. It causes changes in gut microbial profile, reduction of microbial diversity, and the impairment of the intestinal barrier, leading to increased serum lipopolysaccharide (endotoxin) levels. Elevated lipopolysaccharide (LPS) induces acetyltransferase P300 both in the nucleus and cytoplasm of liver hepatocytes through the activation of the IRE1-XBP1 pathway in the endoplasmic reticulum stress. In the nucleus, induced P300 acetylates CRTC2 to increase CRTC2 abundance and drives Foxo1 gene expression, resulting in increased expression of the rate-limiting gluconeogenic gene G6pc and Pck1 and abnormal liver glucose production. Furthermore, abnormal cytoplasm-appearing P300 acetylates IRS1 and IRS2 to disrupt insulin signaling, leading to the prevention of nuclear exclusion and degradation of FOXO1 proteins to further exacerbate the expression of G6pc and Pck1 genes and liver glucose production. Inhibition of P300 acetyltransferase activity by chemical inhibitors improved insulin signaling and alleviated hyperglycemia in obese mice. Thus, P300 acetyltransferase activity appears to be a therapeutic target for the treatment of type 2 diabetes and obesity. Refer to Source Here
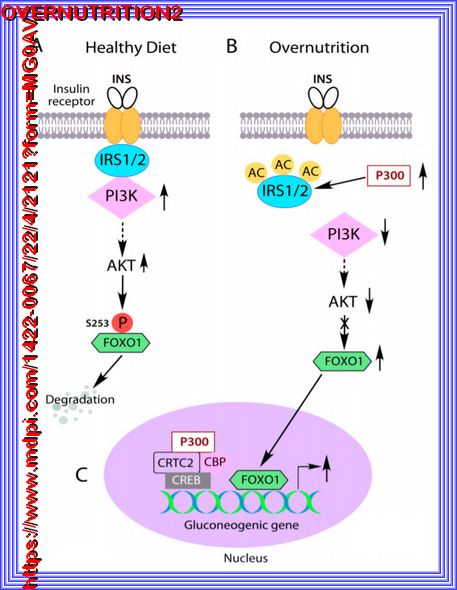
IRS acetylation by abnormal cytoplasm-appearing P300 causes insulin resistance. |
(A) Insulin-mediated activation of PI3K-AKT signaling leads to FOXO1 phosphorylation, nuclear exclusion and degradation, subsequently inhibition of gluconeogenic gene expression in the liver. |
(B) Overnutrition induced abnormal cytoplasm-appearing P300 acetylates IRS1 and IRS2 to disrupt their association with insulin receptors and
insulin signaling. FOXO1 cannot be phosphorylated by AKT |
(C), leading to its nuclear accumulation and stimulation of overexpression of the
gluconeogenic gene in the liver |
(D). The solid arrows indicate the direct effects, the dashed arrows indicate indirect effects, and the crossed line
indicates the blockade of the pathway. |
Overnutrition, particularly a diet high in fructose (from sources like high-fructose corn syrup and sucrose), high glycemic load carbohydrates, and fat should cause a metabolic cascade of: |
---|
1. Lipogenesis: |
2. De Novo Lipolysis: |
3. De Novo Gluconeogenesis as Overnutrition should cause regulation if glycolysis: |
4. Elevated levels of Palmitate: |
5. Hyperglycemia: |
6. Hyperinsulinemia: |
7. Increased levels of P300 in the liver through the leaky gut. |
8. Insulin resistance in the liver directly: |
9. Huge Metabolic problems: |
10. This should cause cytokines to be circulated, IL6 etc |
p300 or CBP is required for insulin-stimulated glucose uptake in skeletal muscle and adipocytes
The carbohydrate-insulin model: a physiological perspective on the obesity pandemic
The Impact of Overnutrition on Insulin Metabolic Signaling in the Heart and the Kidney
Assessing the Nutrient Composition of a Carnivore Diet:
Basic concepts in nutrition: Overnutrition – Functional and clinical consequences
Neuroinflammation and Neurodegeneration in Overnutrition-induced Diseases
METABOLISM
METABOLISM SLIDESHOW
Enzymes: principles and biotechnological applications
The physiological regulation of glucose flux into muscle in vivo
Insulin and β adrenergic receptor signaling: Cross talk in heart
The Multifaceted Pyruvate Metabolism: Role of the Mitochondrial Pyruvate Carrier
Metabolic flexibility and insulin resistance
Glucose transporters: physiological and pathological roles
VIDEO: Steps of Glycolysis Reactions Explained - Animation - SUPER EASY
Importance of GLUT Transporters in Disease Diagnosis and Treatment
Metabolism | The Metabolic Map: Carbohydrates
Metabolism | The Metabolic Map: Lipids
Metabolism | Regulation of Glycolysis
Brain and systemic glucose metabolism in the healthy elderly following fish oil supplementation
Molecular Pathophysiology of Hepatic Glucose Production
REGULATION OF GLUCOSE PRODUCTION BY THE LIVER
Intakes and sources of dietary sugars and their association with metabolic and inflammatory markers
Vitamins and Minerals Involved in Energy Metabolism
Beta-Aminoisobutyric Acid as a Novel Regulator of Carbohydrate and Lipid Metabolism
The Role of Glutathione in Protecting against the Severe Inflammatory Response Triggered by COVID-19
Erythritol Attenuates Postprandial Blood Glucose by Inhibiting α-Glucosidase
D-ALLOUSE Comparison with Fructose and Erythritol
High-Fat Diet or Diabetes Drug May Enhance Response to Targeted Cancer Drug
Carbotoxicity—Noxious Effects of Carbohydrates
Differentiation of Diabetes by Pathophysiology, Natural History, and Prognosis
A Fresh View of Glycolysis and Glucokinase Regulation: History and Current Status*
Fatty acid metabolism, energy expenditure and insulin resistance in muscle
The role of fatty acids in insulin resistance
Evidence for Central Regulation of Glucose Metabolism
Endothelial GLUTs and vascular biology
Neurotransmitters in Type 2 Diabetes and the Control of Systemic and Central Energy Balance
Brain control of blood glucose levels: implications for the pathogenesis of type 2 diabetes
Brain control of blood glucose levels: implications for the pathogenesis of type 2 diabetes
Autonomic control of energy balance and glucose homeostasis
Cardiometabolic multimorbidity, lifestyle behaviours, and cognitive function
Sympathetic nervous system and immune interplay: Key to metabolic regulation
Relationship Between Glucocorticoids and Insulin Resistance in Healthy Individuals
Diabetes and Renin-Angiotensin-Aldosterone System: Pathophysiology and Genetics
Metabolism Mobilization of Triglycerides NN
Glucose Ketone Index (GKI) Calculator
The Randle cycle revisited: a new head for an old hat
The Biochemistry and Physiology of Mitochondrial Fatty Acid β-Oxidation and Its Genetic Disorders
Lipid and glucose metabolism in senescence
Circadian Syndrome Is Associated with Dietary Patterns among Middle-Older Americans
Chemical Reactions in Living Things
Metabolic landscape in cardiac aging: insights into molecular biology and therapeutic implications
Metabolic changes in aging humans: current evidence and therapeutic strategies
METABOLIC FLEXABILITY
OPEN
The Effects of Ketogenic Diet on Insulin Sensitivity and Weight Loss,
Metabolic Flexibility as an Adaptation to Energy Resources and Requirements in Health and Disease
Metabolic Flexibility and Its Impact on Health Outcomes
VIDEO Protein Metabolism Overview, Animation
PI3K-AKT PATHWAY
The PI3K/AKT pathway in obesity and type 2 diabetes
Role of PI3K/AKT Pathway in Insulin-Mediated Glucose Uptake
Insulin–PI3K signalling: an evolutionarily insulated metabolic driver of cancer
Autophagy as an Emerging Target in Cardiorenal Metabolic Disease: from Pathophysiology to Management
Metabolic Role of PTEN in Insulin Signaling and Resistance
Metabolism and proliferation share common regulatory pathways in cancer cells
The PI3K/AKT pathway in obesity and type 2 diabetes.
TCF7L2 regulates pancreatic β-cell function through PI3K/AKT signal pathway
The PTEN–PI3K pathway: of feedbacks and cross-talks
MAPK signal pathways in the regulation of cell proliferation in mammalian cells
Loss of mTORC1 signaling alters pancreatic α cell mass and impairs glucagon secretion
IRS1/PI3K/AKT pathway signal involved in the regulation of glycolipid metabolic abnormalities
Altered Insulin Signaling in Alzheimer’s Disease Brain – Special Emphasis on PI3K-Akt Pathway
The PI3K pathway in human disease
Transcriptional Regulation of INSR, the Insulin Receptor Gene
Signaling pathways in insulin action: molecular targets of insulin resistance
Role of PI3K/AKT Pathway in Insulin-Mediated Glucose Uptake
The role of skeletal muscle Akt in the regulation of muscle mass and glucose homeostasis
An Integrated View of Insulin Resistance and Endothelial Dysfunction
AKT/PKB Signaling: Navigating Downstream
PTEN and the PI3-Kinase Pathway in Cancer
PTEN function, the long and the short of it
Hyperglycemia alters PI3k and Akt signaling
Regulation of PTEN translation by PI3K signaling maintains pathway homeostasis
Management of Phosphatidylinositol-3-Kinase Inhibitor-Associated Hyperglycemia
VIDEO PI3K/Akt pathway - part 5: PTEN
Molecular Targeting of the Phosphoinositide-3-Protein Kinase (PI3K) Pathway across Various Cancers
The PTEN/PI3K/AKT Pathway in vivo, Cancer Mouse Models
Understanding the Warburg Effect: The Metabolic Requirements of Cell Proliferation
The PTEN–PI3K pathway: of feedbacks and cross-talks
PTEN Inhibition in Human Disease Therapy
Metabolic Role of PTEN in Insulin Signaling and Resistance
The Impact of PIK3R1 Mutations and Insulin–PI3K Glycolytic Pathway Regulation in Prostate Cancer
PTEN Mutations as a Cause of Constitutive Insulin Sensitivity and Obesity
https://journals.physiology.org/doi/epdf/10.1152/ajpheart.01088.2004
How does hepatic lipid accumulation lead to lipotoxicity in non-alcoholic fatty liver disease
Regulation of Energy Metabolism by Receptor Tyrosine Kinase Ligands
Targeting PI3K/AKT signaling pathway in obesity
VIDEO SUSANNAH HANNAFORD Insulin PI3K Akt signaling pathway
Role of PI3K/AKT Pathway in Insulin-Mediated Glucose Uptake
3. Role of PI3K/AKT Pathway in Insulin-Mediated Glucose Uptake
The PI3K/AKT pathway in obesity and type 2 diabetes
The Critical Role of Akt in Cardiovascular Function
Molecular Targeting of the Phosphoinositide-3-Protein Kinase (PI3K) Pathway across Various Cancers
Targeting PI3K/Akt signal transduction for cancer therapy
Leucine and mTORc1 act independently to regulate 2-deoxyglucose uptake in L6 myotubes
Amino acid-dependent control of mTORC1 signaling: a variety of regulatory modes
Obesity Alters the Muscle Protein Synthetic Response to Nutrition and Exercise
VIDEO BITTMAN Sarcopenic Obesity
AMPK: Mechanisms of Cellular Energy Sensing and Restoration of Metabolic Balance
mTOR Signaling in Growth, Metabolism, and Disease
Pancreatic β-Cell Electrical Activity and Insulin Secretion: Of Mice and Men
The PI3K-AKT network at the interface of oncogenic signalling and cancer metabolism
AMPK activation can delay aging
AMPK activation/responsiveness decreases with age, resulting in: reduced autophagic clearance of unnecessary products an increase in oxidative stress a decrease resistance to cellular stress
The PI3K/Akt signaling axis in Alzheimer’s disease: a valuable target to stimulate or suppress
The PI3K/AKT pathway in obesity and type 2 diabetes
Role of PI3K/AKT Pathway in Insulin-Mediated Glucose Uptake
The PI3K/Akt Pathway in Meta-Inflammation
The PI3K/AKT pathway in obesity and type 2 diabetes
Role of PI3K/AKT Pathway in Insulin-Mediated Glucose Uptake
I. KREBS CYCLE/NAD
AMPK activation/responsiveness decreases with age, resulting in: reduced autophagic clearance of unnecessary products an increase in oxidative stress a decrease resistance to cellular stress
AMPK: Mechanisms of Cellular Energy Sensing and Restoration of Metabolic Balance
I. KREBS CYCLE/NAD
1. Krebs Cycle or Citric Acid Cycle
2. Krebs / citric acid cycle | Cellular respiration | Biology | Khan Academy
3. TCA/Citric Acid (Krebs) Cycle DIRTY MEDICINE
4. Electron Transport Chain (Oxidative Phosphorylation)
5. Pyruvate Pathways & Metabolism
6. Metabolism - Electron Transport Chain: Overview You Tube
7. Krebs Cycle Reimagined: The Emerging Roles of Succinate and Itaconate as Signal Transducers
Effects of Ketone Bodies on Brain Metabolism and Function in Neurodegenerative Diseases
Mitochondrial pyruvate transport: a historical perspective and future research directions
The role of pyruvate carboxylase in insulin secretion and proliferation in rat pancreatic beta-cells
NADPH and Glutathione Redox Link TCA Cycle Activity to Endoplasmic Reticulum Homeostasis
Citrate – new functions for an old metabolite
ELECTRIC TRANSPORT CHAIN
The Role of Mitochondria in the Pathogenesis of Type 2 Diabetes
Electron Transport Chain (Oxidative Phosphorylation)
BETA OXIDATION
OPEN TEXT
Regulation of Hexokinase Binding to VDAC
Altered Insulin Signaling in Alzheimer’s Disease Brain – Special Emphasis on PI3K-Akt Pathway
IRS1/PI3K/AKT pathway signal involved in the regulation of glycolipid metabolic abnormalities
VIDEO NN - Metabolism | Fatty Acid Oxidation: Part 1 - IRS1/PI3K/AKT pathway
VIDEO NN - Metabolism | Fatty Acid Oxidation: Part 2
VIDEO NN - Metabolism | The Metabolic Map: Proteins
VIDEO NN - Metabolism | Amino Acid Metabolism
NAD+
NAD+
NAD+ in Brain Aging and Neurodegenerative Disorders
ENDOCRINE HORMONES HPA
OPEN TEXT
Hypothalamus-adipose tissue crosstalk: neuropeptide Y and the regulation of energy metabolism
Effect of circadian clock disruption on type 2 diabetes
Thyroid Dysfunction and Diabetes Mellitus: Two Closely Associated Disorders
Effect of circadian clock disruption on type 2 diabetes
Hyperinsulinemia Associated Depression
GLYCOLIPID TOXICITY
Biochemical and major pathways underlying endothelial dysregulation in vascular complications of diabetes. Long-term hyperglycemia and hyperlipidemia can cause endothelial cell dysfunction and increase the adhesion of monocytes and platelets. The former can transform into macrophages, while the latter can recruit blood cells, accumulate in blood vessels, and form thrombi. On the one hand, macrophages invade endothelial cells and engulf ox-LDL, turning into foam cells and forming arterial plaques. Macrophages release inflammatory and transcription factors that aggravate the inflammatory response. Excessive glucose and lipid levels will covalently combine to form AGEs, which can bind to their receptors; activate the MAPK and NF-KB pathways, among others; and reduce the production and utilization of NO. Abnormal glucose and lipid metabolism can also affect mitochondrial function, produce excessive ROS, and lead to insufficient energy supply. Epigenetic modifications are also closely related to vascular injury in the vascular complications of T2D, including histone and DNA modifications, and ncRNA regulation.
The mechanisms of glycolipid metabolism disorder on vascular injury in type 2 diabetes
Cardiac Glucolipotoxicity and Cardiovascular Outcomes
The role of fatty acids in insulin resistance
Fatty acid metabolism, energy expenditure and insulin resistance in muscle
The Randle cycle, the precarious linkbetween sugars and fats
Trapped fat: Obesity pathogenesis as an intrinsic disorder in metabolic fuel partitioning
Chronic inflammation in fat and IR
A nexus of lipid and O-Glcnac metabolism in physiology and disease
Integrating Mechanisms for Insulin Resistance: Common Threads and Missing Links
It Is Not Just Fat: Dissecting the Heterogeneity of Adipose Tissue Function
Adipose tissue plasticity: how fat depots respond differently to pathophysiological cues
LIPOTOXICITY
Increased tissue (muscle and liver) and plasma fat content, i.e., lipotoxicity, plays a central role in the pathogenesis of type 2 diabetes (1,4,8,27,32–34). Elevated bioactive lipids in the circulation, including lipoproteins, triglycerides, and fatty acids (27), and excessive tissue lipid deposits of long-chain fatty acyl CoAs, diacylglycerol, and ceramide (35,36) have been implicated in the phenomenon of lipotoxicity. Much evidence supports a role for circulating free fatty acids in the development of insulin resistance, inflammation, and β-cell dysfunction (1,3,4,33). Recently, elevated plasma sphingolipids have been implicated in the pathogenesis of obesity-induced cardiovascular and metabolic disease (37). Sphingolipid and ceramide formation are stimulated by inflammatory cytokines, such as TNF-α, which is released from adipocytes and elevated in the plasma of type 2 diabetic and obese subjects (5,6). REFER TO THE FIRST REFERENCE BELOW.
Emerging Roles of Ceramide in Cardiovascular Diseases
AMP kinase and malonyl-CoA: Targets for therapy of the metabolic syndrome
Aging adipose: Depot location dictates age-associated expansion and dysfunction
The Transport of Fatty Acids: Roles of Adipose Tissue Proteins
FRUCTOSE
A comparison of the hepatic fructose (left) and glucose (right) metabolism after consumption of high loads of
sugar in the form of SSB. It is hypothesized that an increased de novo lipogenesis after fructose intake in parallel with a decreased
fatty acid oxidation leads to hepatic fat deposition. ACC, acetyl-CoA-carboxylase; ATP, adenosine triphosphate; CPT1a, carnitine palmitoyltransferase
1A; FA, fatty acid; GLUT, glucose transporter; KHK-C, ketohexokinase-C; Ox, oxidation; P, phosphate; SSB, sugar-sweetened beverage; TCA, tricarboxylic acid cycle.
the follow will try to explain the pathophysiology of fructose, considering overnutrition, gluconeogenesis, regulation, etc..
Fructose is a simple sugar (monosaccharide) found in Sucrose, ultra-processed foods, fruits, honey, and high-fructose corn syrup (HFCS).
Its metabolism and pathophysiology differ significantly from glucose, particularly in the context of overnutrition
and metabolic regulation. Below is an explanation of the pathophysiology of fructose, focusing on its metabolism, effects
on gluconeogenesis, and regulatory mechanisms, especially in the setting of excessive intake.
______________________________________
_
1. Fructose Metabolism |
---|
ºFructose is primarily metabolized in the liver, though some metabolism occurs in the intestines and kidneys. The key steps in fructose metabolism are: |
style="border: 3px solid red;">º Absorption: Fructose is absorbed in the small intestine via the GLUT5 transporter. |
º Hepatic metabolism: In the liver, fructose is rapidly phosphorylated by fructokinase to form fructose-1-phosphate (F1P). |
This reaction bypasses the rate-limiting step of glycolysis (phosphofructokinase-1), allowing fructose to enter metabolic pathways more rapidly than glucose. º Cleavage: F1P is cleaved by aldolase B into dihydroxyacetone phosphate (DHAP) and glyceraldehyde, which can enter glycolysis or gluconeogenesis. |
2. Effects of Overnutrition |
º Excessive fructose consumption, particularly from added sugars like HFCS, has been linked to
metabolic dysregulation. Key pathophysiological effects include: |
º Lipogenesis and Fatty Liver |
º Fructose metabolism generates substrates (e.g., acetyl-CoA) that promote de novo lipogenesis (DNL), leading to triglyceride synthesis. |
º This contributes to non-alcoholic fatty liver disease (NAFLD) and hepatic insulin resistance. |
º Fructose does not stimulate insulin secretion directly, but chronic overconsumption leads to: |
º Increased hepatic glucose production. |
º Impaired insulin signaling in peripheral tissues. |
º Systemic insulin resistance, a hallmark of metabolic syndrome. |
º Uric Acid Production |
º Fructose metabolism depletes ATP, leading to increased production of uric acid as a byproduct. |
º Elevated uric acid levels are associated with hypertension, inflammation, and gout. |
º Appetite Dysregulation |
º Fructose does not stimulate leptin (satiety hormone) or suppress ghrelin (hunger hormone) as effectively as glucose. This can lead to overeating and weight gain. |
3. Gluconeogenesis and Fructose |
º Fructose can serve as a substrate for gluconeogenesis, the process by which the liver produces glucose from non-carbohydrate sources. However, excessive fructose intake has paradoxical effects: |
º Increased gluconeogenesis: Fructose provides carbons for glucose synthesis, but overnutrition can lead to excessive glucose production, contributing to hyperglycemia. |
º Dysregulation: Chronic fructose consumption impairs the normal regulation of gluconeogenesis, exacerbating insulin resistance and metabolic dysfunction. |
4. Regulation of Fructose Metabolism |
ºFructose metabolism is less tightly regulated than glucose metabolism, which contributes to its pathophysiological effects: |
º Fructokinase: This enzyme is not regulated by feedback inhibition, allowing uncontrolled entry of fructose into metabolic pathways. |
º Hormonal regulation: Unlike glucose, fructose metabolism is not directly regulated by insulin. However, insulin resistance induced by fructose can indirectly affect its metabolism. |
º AMP deaminase activation: Fructose metabolism depletes ATP, activating AMP deaminase and increasing uric acid production, which further exacerbates metabolic stres |
5. Long-Term Consequences of Excessive Fructose Intake |
ºChronic overconsumption of fructose is associated with: |
º Obesity: Due to its effects on appetite regulation and lipogenesis. |
º Type 2 diabetes: Driven by insulin resistance and impaired glucose homeostasis. |
º Cardiovascular disease: Linked to dyslipidemia, hypertension, and inflammation. |
º NAFLD: Resulting from excessive hepatic lipid accumulation. |
6. Summary Fructose metabolism, while efficient, becomes pathophysiological in the context of overnutrition. Its unregulated entry into metabolic pathways, promotion of lipogenesis, and contribution to insulin resistance and gluconeogenesis dysregulation underlie its role in metabolic syndrome and related disorders. Reducing excessive fructose intake, particularly from added sugars, is crucial for preventing these adverse health outcomes. |
Refer to PMC10488931 FOR DETAILS and (referances). Main pathways of fructose metabolism by the liver. For the sake of clarity, only fructose reactions are shown in this figure, on the understanding that they occur at the same time as glycolysis. The importance of the interactions between glucose and fructose metabolism in the liver is explained in the text, as well as in Figure 3 and Figure 4. More than 90% of absorbed fructose is retained in the liver. Fructose from the portal vein enters the hepatocyte (1) via transporter Glut 2 (a high-capacity transporter also used by glucose) and, secondarily, by Glut 8. The phosphorylation of fructose requires a specific enzyme, either fructokinase or ketohexoquinase C (KHK C) (2). This phosphorylation is very quick and is unregulated (unlike glucose phosphorylation by glucokinase) and may lead to the depletion of ATP if the concentration of fructose is very high (3). Ultimately, the recycling of ADP leads to the production of AMP (4), which is then transformed into uric acid (5). Another enzyme that is specific for the metabolism of fructose is aldolase B (6), which leads to the production of the classic trioses of glycolysis (7) with the aid of triokinase (8). An increase in the flux of trioses may lead, under certain circumstances, to the production of methylglyoxal (9) and advanced glycation end products (10) that damage proteins, lipids, and DNA. Since, usually, fructose is ingested at the same time and in the same amounts as glucose, which is converted largely into glycogen or sent to the circulation, the extra trioses are mostly shunted to acetyl-coA (11) and then enter the pathway for the synthesis of fatty acids, also known as de novo lipogenesis (DNL), via malonyl-coA (12). This molecule commits these carbons to fat as it inhibits CPT1 (13), hindering FA entry into the mitochondria for oxidation. Either by providing the carbons as shown in the figure, or by stimulus (ChREBP and SREBP1c), as discussed in the text and in Figure 3 and Figure 4, fructose provides the trioses for the backbone of triglycerides (TG) and the fatty acids as well (14). TG may accumulate as liver fat (15), or be secreted as VLDL (16) or both, depending on fluxes, other substrates, genetics, and hormones. Overactive DNL, caused by a constant excess of fructose, also leads to the production of ceramides, which may be the basis of hepatic insulin resistance, further complicating the metabolic derangement (17). This figure was partly generated using Servier Medical Art, provided by Servier, licensed under a Creative Commons Attribution 3.0 unported license.
Refer to PMC10488931 FOR DETAILS and (referances). Crosstalk between fructose and glucose metabolism in the liver: the role of F-1-P as a signaling molecule of abundance that has gone awry in current dietary habits. The ingestion of fructose is usually accompanied by a similar amount of glucose, be it in the form of sugar or high-fructose corn syrup. Akin to fructose, glucose is also taken up by Glut 2 transporters in the hepatocytes (1). Fructose-1-P (2), the product of fructokinase, acts as a signaling molecule that activates glucose metabolism by its action on glucokinase, GK (3), and pyruvate kinase (4), resulting in an increased flux of intermediates. After the saturation of glycogenesis (5), these lead to DNL (6) and esterification, with TG as end products (7). TG are packed with apoB100 in VLDL (8) and/or stored as liver fat, as shown in Figure 2. Fructose-1-P induces the expression of hypoxia-inducible factor 1 subunit alpha (HIF1a) (9), which, in turn, promotes microvilli expansion and increased absorptive surface (10), thereby enhancing energy uptake and TG production. This figure was partly generated using Servier Medical Art, provided by Servier, licensed under a Creative Commons Attribution 3.0 unported license.
Fructose is a ketohexose and sweetest among all the natural sugars. Like other reducing sugars, it reacts readily with the amino- and nucleophilic groups of proteins, nucleic acids and other biomolecules resulting in glycation reactions. The non-enzymatic glycation reactions comprise Schiff base formation, their Amadori rearrangement followed by complex and partly incompletely understood reactions culminating in the formation of Advance Glycation End products (AGEs). The AGEs are implicated in complications associated with diabetes, cardiovascular disorders, Parkinson's disease, etc. Fructose is highly reactive and forms glycation products that differ both in structure and reactivity as compared to those formed from glucose. Nearly all tissues of higher organisms utilize fructose but only a few like the ocular lens, peripheral nerves erythrocytes and testis have polyol pathway active for the synthesis of fructose. Fructose levels rarely exceed those of glucose but, in tissues that operate the polyol pathway, its concentration may rise remarkably during diabetes and related disorders. Diet contributes significantly to the body fructose levels however, availability of technologies for the large scale and inexpensive production of fructose, popularity of high fructose syrups as well as the promotion of vegetarianism have resulted in a remarkable increase in the consumption of fructose. In vivo glycation reactions by fructose, therefore, assume remarkable significance. The review, therefore, aims to highlight the uniqueness of glycation reactions with fructose and its role in some pathophysiological situations.
Fructose Metabolism (Fructolysis): Steps and Importance
Hypothesis: Could Excessive Fructose Intake and Uric Acid Cause Type 2 Diabetes?
Biochemistry, Fructose Metabolism
Fructose metabolism and metabolic disease
What’s the Difference Between Fructose and Glucose?
Molecular aspects of fructose metabolism and metabolic disease
Alcoholism and Diabetes Mellitus
Sugar consumption, metabolic disease and obesity: The state of the controversy
Sugar, Uric Acid, and the Etiology of Diabetes and Obesity
Biochemistry, Polyol Or Sorbitol Pathways
Unifying mechanism for diabetic complications
Fructose Metabolism Biochemistry Topics, Carbohydrate Metabolism
Regulation of the fructose transporter GLUT5 in health and disease
Fructose Metabolism and Cardiac Metabolic Stress
Dietary fructose as a metabolic risk factor
Biochemistry, Fructose Metabolism
The sweet path to metabolic demise: fructose and lipid synthesis
Fructose metabolism and metabolic disease
Fructose Metabolism (Fructolysis): Steps and Importance
Fructose Metabolism (Fructolysis): Steps and Importance
Fructose Metabolism (Fructolysis): Steps and Importance
Fructose: A Key Factor in the Development of Metabolic Syndrome and Hypertension
VIDEO: The Dangers of Fructose & Uric Acid - with Dr. Richard Johnson | The Empowering Neurologist
Erythritol Attenuates Postprandial Blood Glucose by Inhibiting α-Glucosidase
D-ALLOUSE Comparison with Fructose and Erythritol
Fructose Impact on Brain Function in Rodent Models
Fructose metabolism and metabolic disease
Endogenous fructose production: what do we know and how relevant is it?
High Fat and High Sucrose (Western) Diet Induce Steatohepatitis that is Dependent on Fructokinase
Added Sugar Intake and Cardiovascular Diseases Mortality Among US Adults
Fructose and NAFLD: The Multifaceted Aspects of Fructose Metabolism
Added Fructose in Non-Alcoholic Fatty Liver Disease and in Metabolic Syndrome:
Fructose as a metabolic toxin that targets the gut-liver axis
High Fructose Intake and Adipogenesis
Fructose, insulin resistance, and metabolic dyslipidemia
Systems Nutrigenomics Reveals Brain Gene Networks Linking Metabolic and Brain Disorders
Chronic Consumption of Fructose Induces Behavioral Alterations by Increasing Orexin and Dopamine
Fructose impairs fat oxidation: Implications for the mechanism of western diet-induced NAFLD
Fructose and Uric Acid: Major Mediators of Cardiovascular Disease Risk Starting at Pediatric Age
Fructose and NAFLD: The Multifaceted Aspects of Fructose Metabolism
High Fructose Intake and Adipogenesis
ChREBP regulates fructose-induced glucose production independently of insulin signaling
Testing the carbohydrate-insulin model in mice:
Dietary fructose enhances tumour growth indirectly via interorgan lipid transfer
Fructose Hijacks the Liver to Grow Cancer
Fructose contributes to the Warburg effect for cancer growth
Recent Progress on Fructose Metabolism—Chrebp, Fructolysis, and Polyol Pathway
Dietary Fructose and Fructose-Induced Pathologies
Fructose drives de novo lipogenesis affecting metabolic health
Sugar and Dyslipidemia: A Double-Hit, Perfect Storm
Role of Dietary Fructose and Hepatic de novo Lipogenesis in Fatty Liver Disease
Sweet but Bitter: Focus on Fructose Impact on Brain Function in Rodent Models
ChREBP regulates fructose-induced glucose production independently of insulin signaling
Fructose and Sugar: A Major Mediator of Nonalcoholic Fatty Liver Disease
Role of Dietary Fructose and Hepatic de novo Lipogenesis in Fatty Liver Disease
Dietary Fructose and the Metabolic Syndrome
A short review on the aetiology and pathophysiology of alcoholism
Alcohol and Metabolic-associated Fatty Liver Disease
GLP-1
Circumventing the issue of cell specificity, is the exploitation of glucagon-like-peptide-1 (GLP-1), as this interacts with a receptor that is only expressed on β-cells (and neurons). GLP-1 is a gut-derived incretin that is naturally produced in response to food ingestion to enhance insulin secretion. Specifically, GLP-1 has been reported to promote proliferation and inhibit apoptosis of β-cells, in vivo and in vitro, and to attenuate apoptosis induced by several pro-apoptotic agents (such as STZ and reactive oxygen species [ROS]).35, 36 Chang et al (2016) showed that GLP-1 suppressed methylglyoxal (MG)-induced apoptosis in a rat insulinoma cell line (RINm5F) by improving mitochondrial function and activating PKA and PI3K/Akt signaling, thereby causing Akt phosphorylation and subsequent inhibition of the cleavage (ie, activation) of pro-apoptotic caspase-3. These effects were corroborated by experiments using MIN6 and INS-1 β-cells.37 In addition to enhancing proliferation and survival, GLP-1 has been recently shown to putatively promote β-cell neogenesis in a severe insulin-deficient diabetic rat model induced by administration of a single high dose of STZ. Indeed, the consequent increase in β-cell number following GLP-1 treatment was not attributable to β-cell replication but rather to α-cell dedifferentiation and subsequent transdifferentiation into glucose-responsive insulin-secreting β-cells. This was associated with the regulation of the GLP-1 receptor and its downstream transcription factor pathway PI3K/Akt/FOXO1.35
While these beneficial effects of GLP-1 strongly support its use as a therapeutic agent, it is rapidly degraded in vivo (t1/2 ~ 2 minutes)
by the endogenous enzyme dipeptidyl-peptidase-IV (DPP-4).38 To extend bioavailability to improve clinical efficacy, several synthetic GLP-1 receptor
agonists have been developed. For instance, the native molecule exendin-4 has been shown to enhance β-cell proliferation and inhibit apoptosis,
both in vitro and in vivo, thereby increasing β-cell number and mass, respectively. These effects were abolished in the presence of the PI3K inhibitor LY294002,
establishing that the pro-survival/proliferation effect was mediated by PI3K/Akt signaling in β-cells.39 Likewise, the human GLP-1 analogue liraglutide
has been shown to promote β-cell proliferation and inhibit apoptosis, both in vitro and in vivo. Indeed, in BTC-6 β-cells, liraglutide suppressed
apoptosis induced by serum withdrawal through PI3K/Akt phosphorylation, leading to the inhibition of caspase-3 activity via a mechanism like that of GLP-1.
The downstream Akt targets, pro-apoptotic Bad and FOXO1 transcription factor, also underwent inhibitory phosphorylation. These observations were corroborated
in an animal model of overt diabetes where liraglutide restored islet size, ameliorated β-cell apoptosis, and increased expression levels of nephrin,
40 a key protein involved in β-cell survival signaling .41 Despite the positive effects on β-cell survival in vivo, there are some concerns
regarding the safety profile of these GLP-1 analogues due to their ability to enhance the proliferation of cells.
While a consensus has not been reached, evidence from some clinical studies has shown an expansion of exocrine and endocrine pancreatic cells
with a possible association to pancreatic cancer.42
As an alternative biologic, γ-aminobutyric acid (GABA) may be a candidate therapeutic agent. It is endogenously produced in β-cells and has
been found to stimulate β-cell proliferation in mouse and human islets through the PI3K/mTORC1 pathway. Co-treatment of mice with both
GABA and Ly49, a novel GABA type A (GABAA) receptor-positive allosteric modulator, amplified these positive effects. Furthermore, co-treatment with
GABA and Ly49 increased β-cell area and proliferation, as compared to mice treated with GABA alone, and similar observations were made using
human islets.43 Importantly, a number of GABAA receptor agonists are currently in clinical use as a treatment for epilepsy,44 which suggests that
they are considered safe for use in humans and so could potentially be tested in clinical trials as a treatment for T1D.
Glucagon-like peptide 1-based therapies for the treatment of type 2 diabetes mellitus
Hyperglycemia Potentiates the Slowing of Gastric Emptying Induced by Exogenous GLP-1
Hyperglycemia Potentiates the Slowing of Gastric Emptying Induced by Exogenous GLP-1
GLP-1 receptor activated insulin secretion from pancreatic β-cells: mechanism and glucose dependence
Diabetes drugs in the GLP-1 agonists
Glucagon-like peptide 1 (GLP-1)
The Physiology of Glucagon-like Peptide 1
Potential preventive properties of GLP-1 receptor agonists against prostate cancer:
ENDIOTHELIAL CELL DYSFUNCTION
Endothelial cells become dysfunctional in high glucose environments, which is connected to glucose metabolism and transport. In normal glucose, endothelial cells take up glucose from the blood via glucose transporters (GLUT) and sodium-glucose cotransporters (SGLT). Glucose can then be metabolized, primarily via glycolysis. Pyruvate is shuttled into the mitochondria to be metabolized in the tricarboxylic acid (TCA) cycle or converted to lactate and transported out of the cell via monocarboxylate transporters (MCT). Alternatively, glucose can be transported to the vessel wall or parenchymal tissue paracellularly through cell–cell junctions or transcellularly via GLUTs and SGLTs at the cell abluminal surface. In high glucose, endothelial cells take up more glucose and increase glucose metabolism via glycolysis. Some of the excess glycolytic intermediate metabolites are shunted down glycolytic side branch pathways that contribute to endothelial dysfunction, including the polyol, pentose phosphate, hexosamine biosynthetic, and methylglyoxal pathways. Side branch pathway metabolism is further increased by superoxide overproduction from excess mitochondrial metabolism. Excess glucose also increases protein kinase C (PKC) activation, changes microRNA (miRNA) and long non-coding RNA (lncRNA) expression, and increases extracellular vesicle (EV) release while changing EV contents.
The Relationship between Erythrocytes and Diabetes Mellitus
Vascular actions of insulin with implications for endothelial dysfunction
Endothelial dysfunction and diabetes: roles of hyperglycemia, impaired insulin signaling and obesity
Role of Lipotoxicity in Endothelial Dysfunction
Role of free fatty acids in endothelial dysfunction
A Narrative Review of Diabetic Macroangiopathy: From Molecular Mechanism to Therapeutic Approaches
Endothelial response to glucose: dysfunction, metabolism, and transport
Vascular endothelial dysfunction, a major mediator in diabetic cardiomyopathy
Endothelial dysfunction in diabetes mellitus
Modulation of endothelium function by fatty acids
Endothelial Toxicity of High Glucose and its by-Products in Diabetic Kidney Disease
Endothelial response to glucose: dysfunction, metabolism, and transport
Reciprocal Relationships Between Insulin Resistance and Endothelial Dysfunction
Endothelial response to glucose: dysfunction, metabolism, and transport
holding
OPEN
The association between blood glucose levels and lipids or lipid ratios in type 2 diabetes patients
Latent autoimmune diabetes in adults (LADA)
Mechanisms of Diabetic Complications
Cognitive decline and dementia in diabetes mellitus: mechanisms and clinical implications
Comprehensive review on the pathogenesis of hypertriglyceridaemia-associated acute pancreatitis
An enzyme that selectively S-nitrosylates proteins to regulate insulin signaling
ADA Differentiation of Diabetes by Pathophysiology, Natural History, and Prognosis
The Ketogenic Effect of Medium-Chain Triacylglycerides
Applications of Medium-Chain Triglycerides in Foods
Effect of circadian clock disruption on type 2 diabetes
Cas9 screens reveal regulators of ageing in neural stem cells
The genetic architecture of protein stability
Mutations that affect protein stability follow simple math rules, study shows
Endocrine role of bone in the regulation of energy metabolism
Exercise-induced exerkines: Secret weapon in disease prevention and therapy
Exercise, exerkines, and cardiometabolic health: from individual players to a team sport
Exerkines’: A Comprehensive Term for the Factors Produced in Response to Exercise
Comprehensive Prognostic Tool for Adults ≥ 70
Obesity and Nonalcoholic Fatty Liver Disease: Biochemical, Metabolic and Clinical Implications
LIVER NAFLD TO DIABETES
Nutrient overload occurs worldwide as a consequence of the modern diet pattern and the physical inactivity that sometimes accompanies it. Cells initiate multiple protective mechanisms to adapt to elevated intracellular metabolites and restore metabolic homeostasis, but irreversible injury to the cells can occur in the event of prolonged nutrient overload. Many studies have advanced the understanding of the different detrimental effects of nutrient overload; however, few reports have made connections and given the full picture of the impact of nutrient overload on cellular metabolism. In this review, detailed changes in metabolic and energy homeostasis caused by chronic nutrient overload, as well as their associations with the development of metabolic disorders, are discussed. Overnutrition-induced changes in key organelles and sensors rewire cellular bioenergetic pathways and facilitate the shift of the metabolic state toward biosynthesis, thereby leading to the onset of various metabolic disorders, which are essentially the downstream manifestations of a misbalanced metabolic equilibrium. Based on these mechanisms, potential therapeutic targets for metabolic disorders and new research directions are proposed.
Overnutrition, particularly a diet high in fructose (from sources like high-fructose corn syrup and sucrose), high glycemic load carbohydrates,
and fat should cause a metabolic cascade of: